Knee laxity refers to excessive looseness or instability of the knee joint, often resulting from ligamentous injury or degeneration. The knee joint relies on ligaments, tendons, and muscles to maintain stability and function. When these structures are compromised, abnormal movement patterns can occur, leading to pain, dysfunction, and an increased risk of further injury.
Understanding knee laxity is critical for orthopedic surgeons, sports medicine specialists, and physiotherapists in diagnosing and managing knee injuries effectively. This article explores the causes, diagnostic techniques, and testing methods for assessing knee laxity.
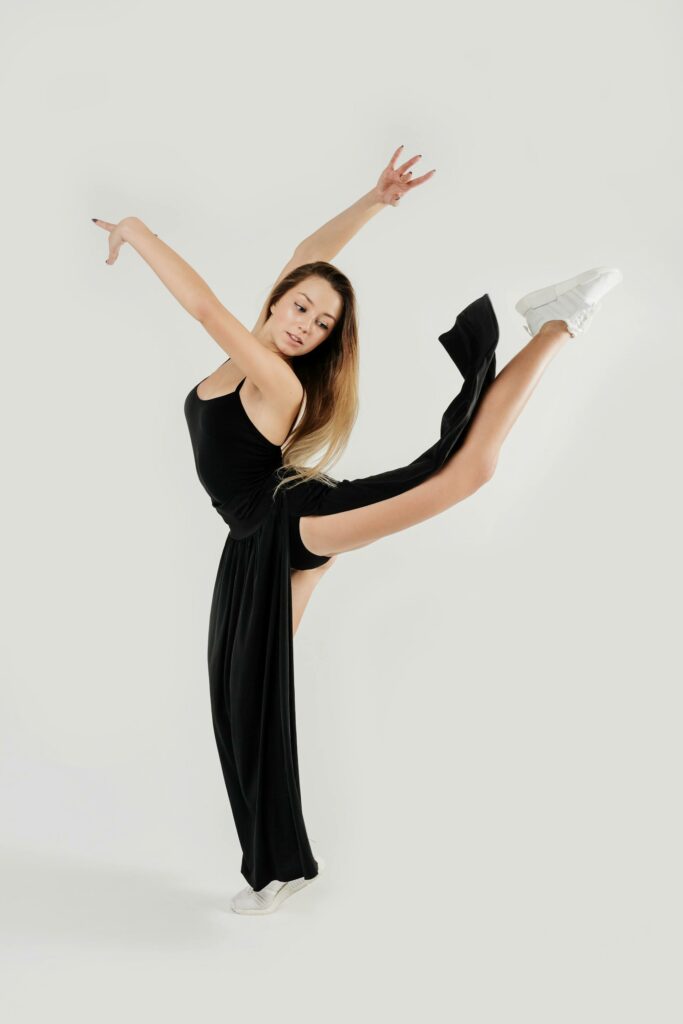
I. Causes of Knee Laxity
Knee laxity can result from traumatic injuries, degenerative conditions, or congenital factors. The primary causes include:
1.1 Ligament Injuries
The anterior cruciate ligament (ACL) and posterior cruciate ligament (PCL) are crucial stabilizers of the knee. Injuries to these ligaments, particularly the ACL, are among the most common causes of knee laxity. ACL ruptures often occur due to sudden deceleration, pivoting, or direct trauma, frequently seen in athletes. The degree of laxity following an ACL tear depends on the extent of ligament damage, the involvement of secondary stabilizers, and the patient’s neuromuscular control.
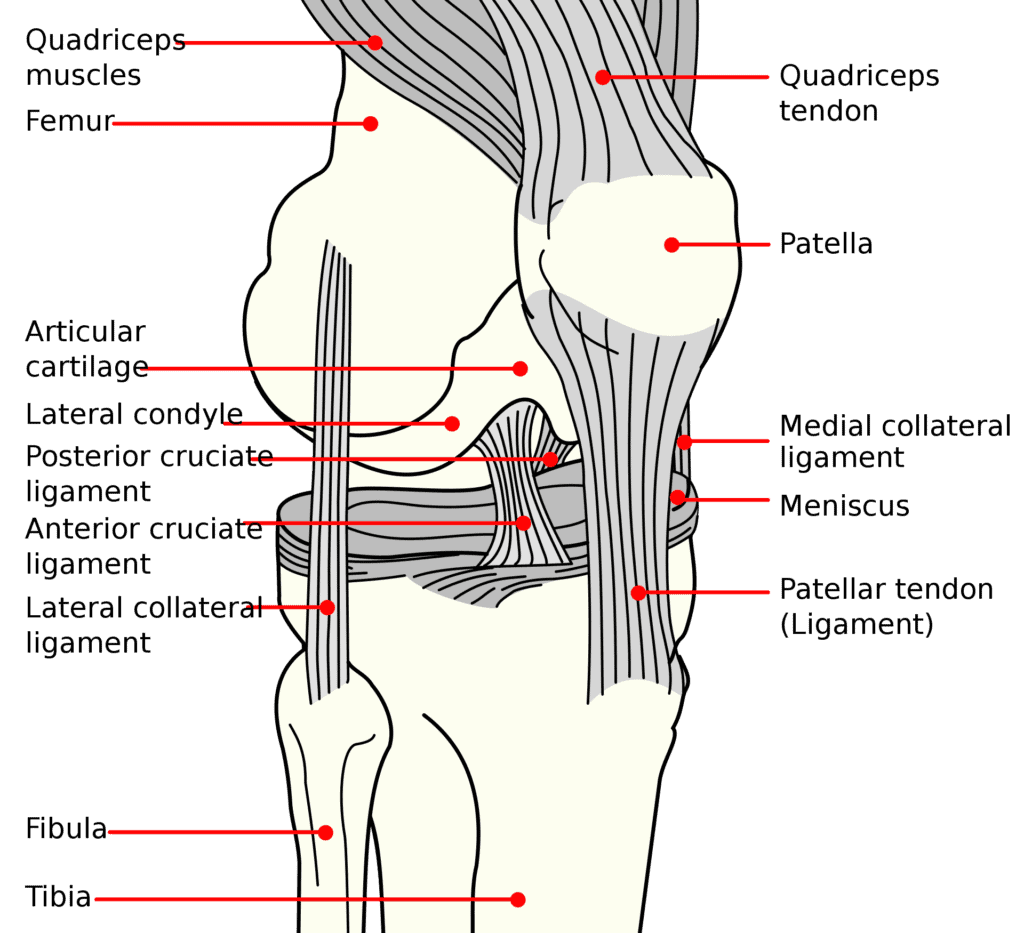
The PCL, though less commonly injured than the ACL, plays a crucial role in preventing posterior tibial translation. PCL injuries typically result from high-energy trauma such as car accidents or direct blows to the anterior tibia. Unlike ACL tears, PCL injuries often allow for greater compensatory mechanisms, reducing the immediate perception of instability.
Similarly, medial collateral ligament (MCL) and lateral collateral ligament (LCL) injuries can contribute to excessive side-to-side motion, further compromising knee stability. The MCL is more frequently injured due to its role in resisting valgus forces, often seen in sports-related contact injuries. Meanwhile, LCL injuries, though less common, can lead to significant lateral knee instability, especially when combined with injuries to the posterolateral corner (PLC) structures.
1.2 Chronic Ligamentous Laxity
Some individuals have generalized joint hypermobility, which predisposes them to increased knee laxity. This condition may be associated with connective tissue disorders such as Ehlers-Danlos Syndrome or Marfan Syndrome. These disorders affect the structure and function of collagen and elastin, the key proteins responsible for ligament strength and flexibility. As a result, individuals with these conditions often exhibit excessive joint motion, frequent dislocations, and a heightened risk of ligament injuries, which can lead to persistent knee instability. Proper diagnosis through genetic testing and clinical assessments is crucial for managing symptoms and preventing further complications.
1.3 Degenerative Changes
With aging or repetitive stress, the knee ligaments may undergo progressive weakening and elongation, leading to instability. This process is often exacerbated by reduced collagen production and decreased tensile strength of ligamentous tissues. Additionally, prolonged mechanical overload and altered joint biomechanics can contribute to ligament insufficiency.
Osteoarthritis (OA) plays a significant role in knee laxity by causing progressive cartilage degradation, osteophyte formation, and synovial inflammation, all of which lead to joint instability. As the protective cartilage deteriorates, the underlying bone surfaces become exposed, increasing friction and further destabilizing the joint. This progressive cycle of degeneration can result in pain, joint misalignment, and functional impairment, making early detection and intervention crucial for maintaining knee function and delaying the need for surgical interventions such as total knee replacement.
1.4 Post-Surgical or Iatrogenic Causes
Surgical procedures such as meniscectomies or poorly executed ligament reconstructions can lead to residual knee laxity. Improper graft tensioning during ACL reconstruction may result in persistent instability, which can be further exacerbated by incorrect tunnel placement, inadequate graft fixation, or insufficient post-operative rehabilitation. Additionally, factors such as graft choice (autograft vs. allograft), biological integration, and patient adherence to recovery protocols play crucial roles in determining long-term knee stability. In cases where residual laxity persists despite surgical intervention, revision procedures, ligament augmentation, or bracing strategies may be necessary to restore functional stability.
II. Symptoms of Increased Knee Laxity
Knee laxity can manifest in various ways depending on the severity of ligament damage and underlying conditions. Common symptoms include:
Knee instability – A feeling of the knee giving way, especially during movement or weight-bearing activities.
Pain and discomfort – Varying from mild to severe, particularly after physical activity.
Swelling – Often occurs after ligament injury due to inflammation and joint fluid accumulation.
Limited range of motion – Stiffness or difficulty in fully extending or flexing the knee.
Clicking or popping sensations – Audible or felt within the joint, indicating ligament or meniscal involvement.
Weakness or muscle atrophy – Reduced muscle strength due to prolonged instability or compensatory movement patterns.
III. Diagnosis of Knee Laxity
A comprehensive clinical assessment, imaging, and biomechanical testing are essential for diagnosing knee laxity accurately.
3.1 Clinical Examination
Orthopedic specialists perform manual stability tests to evaluate knee laxity. The most commonly used clinical tests include:
Lachman Test – Assesses anterior translation of the tibia relative to the femur, primarily testing ACL integrity.
Anterior Drawer Test – Another test for ACL laxity, though less sensitive than the Lachman test.
Pivot Shift Test – Evaluates rotational instability in ACL-deficient knees.
Posterior Drawer Test – Assesses PCL laxity.
Varus and Valgus Stress Tests – Determine the integrity of the MCL and LCL.
3.2 Imaging Techniques
Imaging plays a crucial role in confirming ligamentous injuries and associated knee pathologies:
Magnetic Resonance Imaging (MRI) – The gold standard for detecting ligament tears, meniscal injuries, and cartilage damage.
X-rays with Stress Views – Can provide indirect evidence of ligament insufficiency by showing abnormal joint gapping.
Ultrasound – Useful for assessing collateral ligament injuries in real-time.
3.3 Objective Robotic Arthrometry Testing
Advanced biomechanical testing offers quantitative and reproducible assessments of knee laxity, enabling clinicians to obtain precise data on ligament stability. Recent studies have demonstrated the clinical value of robotic arthrometry in different aspects of knee assessment:
- Diagnosis of ACL injuries: Cojean et al. (2023) conducted a prospective diagnostic study demonstrating that the GNRB laximeter provides sensitivity and specificity comparable to MRI in detecting partial and complete ACL tears, confirming its reliability as a complementary diagnostic tool. DOI: 10.1016/j.knee.2023.03.017
Surgical Planning: Guegan et al. (2024) utilized the DYNEELAX® laximeter in a cadaveric study, highlighting the role of menisco-ligamentary structures in knee stability. Their findings emphasized the importance of individualized surgical planning based on biomechanical data. DOI: 10.1002/jeo2.12038
Post-Surgical Graft Monitoring: Pouderoux et al. (2019) investigated joint laxity evolution after ACL reconstruction using the GNRB arthrometer. Their study revealed that graft compliance and laxity increase during the first postoperative year, underscoring the necessity for personalized rehabilitation protocols. DOI: 10.1007/s00167-019-05711-z
Robotic arthrometers such as DYNEELAX® and GNRB® provide detailed, objective measurements by evaluating anterior tibial translation under controlled and repeatable forces, minimizing the variability inherent in manual testing. These devices are particularly useful in distinguishing between partial and complete ligamentous insufficiency, as well as tracking progression over time in patients undergoing conservative treatment or post-surgical rehabilitation.
Beyond diagnostic capabilities, robotic arthrometry is also instrumental in treatment decision-making. It allows surgeons to assess pre-operative laxity, determine appropriate surgical techniques, and confirm post-operative graft stability. In rehabilitation settings, these devices assist physiotherapists in monitoring patient recovery, tailoring rehabilitation protocols, and ensuring optimal functional outcomes. As a result, robotic arthrometry is increasingly recognized as an essential tool in evidence-based orthopedic and sports medicine practice.
3.4 Manual Arthrometers
Instrumented arthrometers, such as the KT-1000 and KT-2000, were historically used for quantitative ACL laxity assessment. However, these devices have limitations in standardization and accuracy compared to modern robotic systems. A comparative study by Collette et al. (2012) demonstrated that the GNRB arthrometer provides superior intra- and inter-examiner reproducibility compared to the KT-1000, highlighting its advantages in precise anterior knee laxity measurements. DOI: 10.1007/s00167-011-1869-2
Conlusion
Knee laxity is a critical concern in orthopedics, requiring accurate diagnosis and reliable testing methods. While traditional clinical and imaging techniques remain essential, the evolution of robotic arthrometry has revolutionized the field, providing precise and objective assessments of ligament stability.
For healthcare professionals involved in sports medicine, orthopedic surgery, and rehabilitation, utilizing advanced testing tools like DYNEELAX® and GNRB® can significantly enhance patient outcomes by facilitating early diagnosis, tailored treatment plans, and post-surgical monitoring.
References
Cojean et al. (2023) – GNRB laximeter with magnetic resonance imaging in clinical practice for complete and partial anterior cruciate ligament tears detection: A prospective diagnostic study with arthroscopic validation on 214 patients. Knee. DOI: 10.1016/j.knee.2023.03.017
Guegan et al. (2024) – All the menisco‐ligamentary structures of the medial plane play a significant role in controlling anterior tibial translation and tibial rotation of the knee: Cadaveric study of 29 knees with the Dyneelax® laximeter. Journal of Experimental Orthopaedics. DOI: 10.1002/jeo2.12038
Pouderoux et al. (2019) – Joint laxity and graft compliance increase during the first postoperative year after ACL reconstruction: A longitudinal analysis using the GNRB arthrometer. Knee Surgery, Sports Traumatology, Arthroscopy. DOI: 10.1007/s00167-019-05711-z
Collette et al. (2012) – Objective evaluation of anterior knee laxity: Comparison of the KT-1000 and GNRB arthrometers. Knee Surgery, Sports Traumatology, Arthroscopy. DOI: 10.1007/s00167-011-1869-2